Physicists set new record for
quantum memory storage
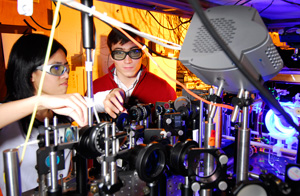
Ran Zhao and Yaroslav Dudin, graduate students in the Georgia
Tech School of Physics, adjust optics in a system used to study
quantum memory. (c) Georgia Tech Photo: Gary Meek
Physicists have taken a significant step toward creation of
quantum networks by establishing a new record for the length of
time that quantum information can be stored in and retrieved
from an ensemble of very cold atoms. Though the information
remains usable for just milliseconds, even that short lifetime
should be enough to allow transmission of data from one quantum
repeater to another on an optical network.
The new record - 7 milliseconds for rubidium atoms stored in a
dipole optical trap - is reported 7 December in the online
version of the journal Nature Physics by researchers at the
Georgia Institute of Technology. The previous record for storage
time was 32 microseconds, a difference of more than two orders
of magnitude.
'This is a really significant step for us, because conceptually
it allows long memory times necessary for long-distance quantum
networking,' said Alex Kuzmich, associate professor in the
Georgia Tech School of Physics and a co-author of the paper.
'For multiple architectures with many memory elements, several
milliseconds would allow the movement of light across a thousand
kilometres.'
The keys to extending the storage time included the use of a
one-dimensional optical lattice to help confine the atoms and
selection of an atomic phase that is insensitive to magnetic
effects. The research was sponsored by the National Science
Foundation, the A.P. Sloan Foundation and the U.S. Office of
Naval Research.
The general purpose of quantum networking or quantum computing
is to distribute entangled qubits - two correlated data bits
that are either '0' or '1' - over long distances. The qubits
would travel as photons across existing optical networks that
are part of the global telecommunications system.
Because of loss in the optical fibre that makes up networks,
repeaters must be installed at regular intervals - about every
100 kilometres - to boost the signal. Those repeaters will need
quantum memory to receive the photonic signal, store it briefly
and then produce a photonic signal that will carry the
information to the next node, and on to its final destination.
For their memory, the Georgia Tech researchers used an ensemble
of rubidium-87 atoms that is cooled to almost absolute zero to
minimise atomic motion. To store information, the entire atomic
ensemble is exposed to laser light carrying a signal, which
allows each atom to participate in the storage as part of a
'collective excitation.'
In simple terms, each atom 'sees' the incoming signal - which is
a rapidly oscillating electromagnetic field - slightly
differently. Each atom is therefore imprinted with phase
information that can later be 'read' from the ensemble with
another laser.
Even though they are very cold, the atoms of the ensemble are
free to move in a random way. Because each atom stores a portion
of the quantum information and that data's usefulness depends on
each atom's location in reference to other atoms, significant
movement of the atoms could destroy the information.
'The challenge for us in implementing these long-lived quantum
memories is to preserve the phase imprinting in the atomic
ensemble for as long as possible,' explained Stewart Jenkins, a
School of Physics research scientist who participated in the
research. 'It turns out that is difficult to do experimentally.'
To extend the lifetime of their memory, the Georgia Tech
researchers took two approaches. The first was to confine the
atoms using an optical lattice composed of laser beams. Because
of the laser frequencies chosen, the atoms are attracted to
specific locations within the lattice, though they are not held
tightly in place.
Because the ensemble atoms are affected by environmental
conditions such as magnetism, the second strategy was to use
atoms that had been pumped to the so-called 'clock transition
state' that is relatively insensitive to magnetic fields.
'The most critical aspect to getting these long coherence times
was the optical lattice,' Jenkins explained. 'Although atoms had
been confined in optical lattices before, what we did was to use
this tool in the context of implementing quantum memory.'
Other research teams have stored quantum information in single
atoms or ions. This simpler approach allows longer storage
periods, but has limitations, he said.
'The advantage of using these ensembles as opposed to single
atoms is that if we shine into them a 'read' laser field,
because these atoms have a particular phase imprinted on them,
we know with a high degree of probability that we are going to
get a second photon - the idler photon - coming out in a
particular direction,' Jenkins explained. 'That allows us to put
a detector in the right location to read the photon.'
Though the work significantly advances quantum memories,
practical quantum networks probably are at least a decade away,
Kuzmich believes.
'In practice, you will need to make robust repeater nodes with
hundreds of memory elements that can be quickly manipulated and
coupled to the fiber,' he said. 'There is likely to be slow
progress in this area with researchers gaining better and better
control of quantum systems. Eventually, they will get good
enough so we can make a jump to having systems that can work
outside the laboratory environment.'
In addition to Kuzmich and Jenkins, the research team included
Ran Zhao, Yaroslav Dudin, Corey Campbell, Dzmitry Matsukevich,
and Brian Kennedy, a professor in the School of Physics.
Source: Georgia
Institute of Technology Research News