Elusive Higgs Boson May Nearly Be
Cornered
ScienceDaily (Mar. 7, 2012) —
New measurements announced March 7 by scientists
from the CDF and DZero collaborations at the U.S.
Department of Energy's Fermi National Accelerator
Laboratory indicate that the elusive Higgs boson may
nearly be cornered. After analyzing the full data
set from the Tevatron accelerator, which completed
its last run in September 2011, the two independent
experiments see hints of a Higgs boson.
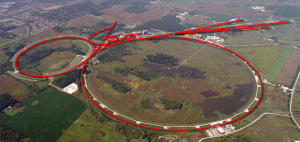
he 4-mile in circumference Tevatron accelerator at
Fermilab uses superconducting magnets chilled to
minus 450 degrees Fahrenheit, as cold as outer
space, to move particles at nearly the speed of
light. (Credit: Image courtesy of DOE/Fermi National
Accelerator Laboratory)
Physicists from the CDF and DZero collaborations
found excesses in their data that might be
interpreted as coming from a Higgs boson with a mass
in the region of 115 to 135 GeV. In this range, the
new result has a probability of being due to a
statistical fluctuation at level of significance
known among scientists as 2.2 sigma. This new result
also excludes the possibility of the Higgs having a
mass in the range from 147 to 179 GeV.
Physicists claim evidence of a new particle only if
the probability that the data could be due to a
statistical fluctuation is less than 1 in 740, or
three sigmas. A discovery is claimed only if that
probability is less than 1 in 3.5 million, or five
sigmas.
This result sits well within the stringent
constraints established by earlier direct and
indirect measurements made by CERN's Large Hadron
Collider, the Tevatron, and other accelerators,
which place the mass of the Higgs boson within the
range of 115 to 127 GeV. These findings are also
consistent with the December 2011 announcement of
excesses seen in that range by LHC experiments,
which searched for the Higgs in different decay
patterns. None of the hints announced so far from
the Tevatron or LHC experiments, however, are strong
enough to claim evidence for the Higgs boson.
"The end game is approaching in the hunt for the
Higgs boson," said Jim Siegrist, DOE Associate
Director of Science for High Energy Physics. "This
is an important milestone for the Tevatron
experiments, and demonstrates the continuing
importance of independent measurements in the quest
to understand the building blocks of nature."
Physicists from the CDF and DZero experiments made
the announcement at the annual conference on
Electroweak Interactions and Unified Theories known
as Rencontres de Moriond in Italy. This is the
latest result in a decade-long search by teams of
physicists at the Tevatron.
"I am thrilled with the pace of progress in the hunt
for the Higgs boson. CDF and DZero scientists from
around the world have pulled out all the stops to
reach this very nice and important contribution to
the Higgs boson search," said Fermilab Director Pier
Oddone. "The two collaborations independently combed
through hundreds of trillions of proton-antiproton
collisions recorded by their experiments to arrive
at this exciting result."
Higgs bosons, if they exist, are short-lived and can
decay in many different ways. Just as a vending
machine might return the same amount of change using
different combinations of coins, the Higgs can decay
into different combinations of particles.
Discovering the Higgs boson relies on observing a
statistically significant excess of the particles
into which the Higgs decays and those particles must
have corresponding kinematic properties that allow
for the mass of the Higgs to be reconstructed.
"There is still much work ahead before the
scientific community can say for sure whether the
Higgs boson exists," said Dmitri Denisov, DZero
co-spokesperson and physicist at Fermilab. "Based on
these exciting hints, we are working as quickly as
possible to further improve our analysis methods and
squeeze the last ounce out of Tevatron data."
Only high-energy particle colliders such as the
Tevatron and LHC can recreate the energy conditions
found in the universe shortly after the Big Bang.
According to the Standard Model, the theory that
explains and predicts how nature's building blocks
behave and interact with each other, the Higgs boson
gives mass to other particles.
"Without something like the Higgs boson giving
fundamental particles mass, the whole world around
us would be very different from what we see today,"
said Giovanni Punzi, CDF co-spokesperson and
physicist at the National Institute of Nuclear
Physics, or INFN, in Pisa, Italy. "Physicists have
known for a long time that the Higgs or something
like it must exist, and we are eager to finally pin
this phenomenon down and start learning more about
it."
If a Higgs boson is created in a high-energy
particle collision, it immediately decays into
lighter more stable particles before even the
world's best detectors and fastest computers can
snap a picture of it. To find the Higgs boson,
physicists retraced the path of these secondary
particles and ruled out processes that mimic its
signal.
The experiments at the Tevatron and the LHC offer a
complementary search strategy for the Higgs boson.
The Tevatron was a proton/anti-proton collider, with
a maximum center of mass energy of 2 TeV, whereas
the LHC is a proton/proton collider that will
ultimately reach 14 TeV. Because the two
accelerators collide different pairs of particles at
different energies and produce different types of
backgrounds, the search strategies are different. At
the Tevatron, for example, the most powerful method
is to search the CDF and DZero datasets to look for
a Higgs boson that decays into a pair of bottom
quarks if the Higgs boson mass is approximately
115-130 GeV. It is crucial to observe the Higgs
boson in several types of decay modes because the
Standard Model predicts different branching ratios
for different decay modes. If these ratios are
observed, then this is experimental confirmation of
both the Standard Model and the Higgs.
"The search for the Higgs boson by the Tevatron and
LHC experiments is like two people taking a picture
of a park from different vantage points," said
Gregorio Bernardi, DZero co-spokesperson at the
Nuclear Physics Laboratory of the High Energies, or
LPNHE, in Paris . "One picture may show a child that
is blocked from the other's view by a tree. Both
pictures may show the child but only one can resolve
the child's features. You need to combine both
viewpoints to get a true picture of who is in the
park. At this point both pictures are fuzzy and we
think maybe they show someone in the park.
Eventually the LHC with future data samples will be
able to give us a sharp picture of what is there.
The Tevatron by further improving its analyses will
also sharpen the picture which is emerging today."
This new updated analysis uses 10 inverse femtobarns
of data from both CDF and DZero, the full data set
collected from 10 years of the Tevatron's collider
program. Ten inverse femtobarns of data represents
about 500 trillion proton-antiproton collisions.
Data analysis will continue at both experiments.
"This result represents years of work from hundreds
of scientists around the world," said Rob Roser, CDF
co-spokesperson and physicist at Fermilab. "But we
are not done yet -- together with our LHC
colleagues, we expect 2012 to be the year we know
whether the Higgs exists or not, and assuming it is
discovered, we will have first indications that it
behaves as predicted by the Standard Model."
Source: Sience
Daily
|