A model burster
Researchers find
the first neutron star that bursts as predicted.
Jennifer Chu, MIT News
Office
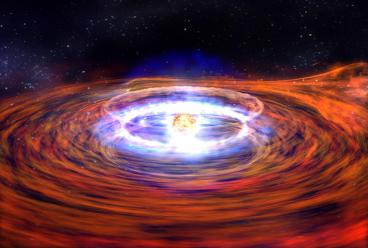
Plasma from a neighboring star gets pulled into
the orbit of a neutron star, where it slams into the stellar
surface, creating thermonuclear explosions.
Image: NASA
For the first time, researchers at MIT and
elsewhere have detected all phases of thermonuclear burning in a
neutron star. The star, located close to the center of the
galaxy in the globular cluster Terzan 5, is a “model burster,”
says Manuel Linares, a postdoc at MIT’s Kavli Institute for
Astrophysics and Space Research.
Linares and his colleagues from MIT, McGill
University, the University of Minnesota and the University of
Amsterdam analyzed X-ray observations from NASA’s Rossi X-ray
Timing Explorer (RXTE) satellite, and discovered the star is the
first of its kind to burst the way that models predict. What’s
more, the discovery may help explain why such a model star has
not been detected until now. A paper to be published in the
March 20 issue of The
Astrophysical Journal details
the group’s findings.
“These are extreme laboratories,” Linares says.
“We can study fundamental physics by looking at what happens on
and around the surface of neutron stars.”
A white-hot environment
Neutron stars typically arise from the collapse
of massive stars. These stellar remnants are made almost
entirely of neutrons, and are incredibly dense — about the mass
of the sun, but squeezed into a sphere just a few miles wide.
For the past three decades, astrophysicists have studied neutron
stars to understand how ultradense matter behaves.
In particular, researchers have focused on the
extremely volatile surfaces of neutron stars. In a process
called accretion, white-hot plasma pulled from a neighboring
star rains down on the surface of a neutron star with incredible
force — equivalent to 100 kilograms (220 pounds) of matter
slamming into an area the size of a coin every second. As more
plasma falls, it forms a layer of fuel on the neutron star’s
surface that builds to a certain level, then explodes in a
thermonuclear fusion reaction. This explosion can be detected as
X-rays in space: The bigger the explosion, the greater the X-ray
intensity, which can be measured as a spike in satellite data.
Researchers have developed models to predict how
a neutron star should burst, based on how much plasma the star
is attracting to its surface. For example, as more and more
plasma falls on a neutron star, explosions should occur more
frequently, resulting in more X-ray spikes. Models have
predicted that at the highest mass-accretion rates, plasma falls
at such a high rate that thermonuclear fusion is stable, and
occurs continuously, without giant explosions.
However, in the last several decades, X-ray
observations from nearly 100 exploding neutron stars have failed
to validate these theoretical predictions.
“Since the late 1970s, we mostly saw bursts at
low mass-accretion rates, and few or no bursts at all at high
mass-accretion rates,” Linares says. “It should be happening,
but for three decades, we didn’t see it. That’s the puzzle.”
Spikes in the data
In late 2010, the RXTE satellite detected X-ray
spikes from a binary star system — two stars bound by gravity
and orbiting close to each other — in Terzan 5. Linares and his
colleagues obtained data from the satellite and analyzed the
data for characteristic spikes.
The team found the system’s neutron star indeed
exhibited X-ray patterns consistent with low mass-accretion
rates, in which plasma fell to the surface slowly. These
patterns looked like large spikes in the data, separated by long
periods of little activity.
To their surprise, the researchers found evidence
for higher mass-accretion rates, where more plasma falls more
frequently — but in these cases, the X-ray data showed smaller
spikes, spaced much closer together. Even higher still, the data
seemed to even out, looking more like an oscillating wave.
Linares interpreted this last observation as a sign of
marginally stable burning: a stage where a neutron star attracts
plasma to its surface at such a high rate that nuclear fusion
reactions take place evenly throughout the plasma layer, without
exhibiting large explosions or spikes.
“We saw exactly the evolution that theory
predicts, for the first time,” says Deepto Chakrabarty,
professor of physics at MIT, and a member of the research team.
“But the question is, why didn’t we see that before?”
Turn, turn, turn
The team soon identified a possible explanation
by comparing the neutron star with others that have been studied
in the past. The one big difference they found was that the
neutron star in question exhibited a much slower rate of
rotation. While most neutron stars rotate a dizzying 200 to 600
times per second, this new star rotated much more slowly, at 11
rotations per second.
The group reasoned that in predicting bursting
behavior, existing models have failed to account for a star’s
period of rotation. The reason this new star matches models so
well, Linares says, is because its rate of rotation is almost
negligible.
It’s still unclear exactly how rotation affects
thermonuclear burning, although Linares has a hunch: Rotation
can cause friction between layers of plasma and a neutron star’s
surface. This friction can release heat, which in turn can
affect the rate of nuclear burning.
“That’s something that we need to look into,”
Linares says. “And now models will have to incorporate rotation,
and will have to explain exactly how that physics works.”
Coleman Miller, professor of astronomy at the
University of Maryland, agrees that rotation may be the most
significant factor that models have overlooked. However, he says
designing models with rotation in mind is an incredibly
data-intensive feat, since thermonuclear fusion often occurs
incredibly quickly, in tiny pockets of a neutron star.
“If you’re going to fully model out a burst, you
have to resolve microseconds and centimeters,” says Miller, who
did not take part in the research. “No computer has been
designed to do this. So these are interesting, likely
suggestions, but it is going to be profoundly difficult to
confirm in a definitive way.
Source: MIT
1 2 3 4 5 6 7 8 9 10 Newest
articles
|