BIG BANG UNDERGROUND
May 25 2008
A 27-km
underground loop of magnets beneath a tranquil patch of
farmland in Europe will soon go to work on the
universe’s deepest mysteries.
For a research
physicist, Sandra Ciocio knows all about the trials of
the construction site. In recent years she’s seen 7,000
tons of sensitive equipment lowered down a 100-m shaft
to prepare for a single grand experiment. The technology
was groundbreaking and the schedule punishing. “It’s
been deadline, deadline, deadline,” she says. “I haven’t
had a holiday in five years.” But when the first real
data begin to emerge this summer, the possible rewards
should be worth the effort: a final explanation of one
of the last puzzles of physics. “I feel like crying,”
says Ciocio. “It’s like a dream come true.”
Deep beneath a tranquil patch of farmland, Ciocio and
her colleagues at the European Organization for Nuclear
Research, known as CERN, have built the world’s biggest
and most sophisticated scientific instrument, the Large
Hadron Collider, housed in a 27-kilometer tunnel that
loops beneath the French-Swiss border. Using
unprecedented energy, it will re-create the conditions a
fraction of a millisecond after the big bang that gave
birth to the cosmos 14 billion years ago. The goal: to
track down a single elusive particle whose existence —
if it can be proved — would fill a critical gap in our
understanding of the universe.
This particular mystery has a daunting history. More
than 30 years ago scientists developed an elegant series
of equations, called the Standard Model, that describes
the make-up of the universe in terms of the relationship
between a few fundamental particles and forces. But the
model has gaps. One gap is the baffling issue of mass.
Why are some particles heavy while others have no mass
at all? According to the leading theory, the weight of a
particle depends on how it interacts with a mysterious
“Higgs field” that permeates all space. So far
scientists haven’t found any evidence that this field —
and its associated particle, the Higgs boson — exists.
They’ve been waiting for a particle collider big enough
to perform the necessary experiments. The Large Hadron
Collider was built to fit this bill.
The idea behind the collider is simple: get protons —
positively charged particles present in every atom —
going fast, crash them into each other and observe the
fragments. The LHC will use superconducting magnets to
guide the protons round and round the subterranean ring
until they’re going almost as fast as light. The
resulting collisions will release unprecedented amounts
of energy (equivalent to 100,000 times the temperature
at the center of the sun). With luck, they’ll also
produce, among a shower of lesser particles, the
long-sought Higgs boson.
The collider may
also throw up clues to puzzles that arise at the strange
intersection of particle physics and astronomy. To
understand the cosmos, scientists must understand how it
developed from those first primordial particles. “In
effect, what we have is far and away the most capable
microscope ever built, and the most powerful telescope
ever built,” says theoretical physicist John Ellis. A
central mystery is the supposed existence of invisible
“dark matter,” and its counterpart “dark energy,” a
strange force that seems to accelerate the expansion of
the universe. Although together the dark pair make up
for 96 percent of the universe, scientists know next to
nothing about them — only their gravitational effects.
Those grand collisions may produce undiscovered
particles that account for both. The collider might also
reveal yet another set of particles, the
“superpartners,” needed to bolster the case for String
Theory, a “theory of everything” that proposes the
existence of six extra dimensions and a universe
constructed of tiny vibrating strings.
All this knowledge comes with a whopping price tag: the
collider will cost about euro 3 billion. Its annual
energy consumption will match the entire city of
Geneva’s. The 1 billion collisions taking place every
second, captured and filtered by underground detectors,
will generate enough data to fill 100,000 CDs a year.
But ultimate knowledge is worth it, says CERN boss
Robert Aymar.
It’s entirely possible that after all this money and
effort the collider’s detectors will find no trace of
the Higgs boson. That would still make the project
worthwhile, researchers say. It would indicate beyond
doubt that the Standard Model, the basis of modern
physics, requires a radical rethinking. “Our theorist
friends tell us to look this way or the other, but maybe
Nature is telling us to look behind us,” says Tejinder
Virdee, a physicist from Imperial College in London.
The Geneva experiments will keep scientists occupied for
20 years or more. “This is truly a once-in-a-generation
experiment,” says Virdee, “but it will take a generation
to do.” Setting up the world’s greatest experiment took
more than a decade: fixing the nature of the cosmos will
take a little longer.
-WILLIAM UNDERHILL (Newsweek)
editor@expressindia.com
Source
http://www.indianexpress.com/sunday/story/304922.html
Scientist Hope to Unlock Scientific Mysteries
with World's Most Powerful Subatomic Collider
When the world's
most powerful subatomic particle collider begins gathering data
this summer, it will be a major milestone for a number ofUniversity
of Washington scientists.
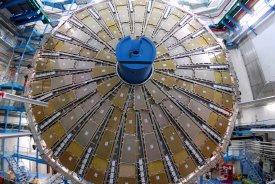
The UW, led by
professors Henry Lubatti in physics and Colin Daly in mechanical
engineering, played a central role in designing and fabricating
nearly 90,000 tubes that are key to the workings of the Atlas
detector. Atlas is one of six particle physics experiments that
are part of the Large Hadron Collider at the European
Organization for Nuclear Research, known as CERN, near Geneva,
Switzerland.
Physicists the world over are
hoping that Atlas will help unlock some deep scientific
mysteries and perhaps even lead to discovery of the Higgs boson,
sometimes called "the God particle" because it is believed its
discovery will refine the understanding of exactly how the
universe came to be and how it functions, and how mass came to
be in the first place.
UW researchers are primarily
involved with an Atlas subsystem that detects subatomic
particles called muons. These particles have little interaction
with each other or with other matter and are formed as a
byproduct of the collisions between protons, the nuclei of
hydrogen atoms. The collider will provide far too much data for
scientists to log all of it, so the first appearance of muons
can be a signal that scientists need to record information on
collisions taking place at that time.
"They are like little messengers
that tell us a potentially interesting event may have occurred,
a signal that we should look more closely at that event,"
Lubatti said.
Potentially that could lead to
direct evidence of the elusive Higgs boson.
"That's just one example of the
detector's value," Lubatti added. "There are many other
interactions that produce high-energy muons, so it is very
important to be able to observe these."
The scientists are looking for
other information that will help them to fill gaps in what they
call the Standard Model of particle physics, a framework that
explains the fundamental forces of nature. The Standard Model
explains the way particle interactions create the strong nuclear
force, the electroweak force and electromagnetism, and how those
forces work with each other, but aspects of those interactions
still are not well understood. The Large Hadron Collider also
could lead to better understanding of the fourth fundamental
force – gravity – in terms of particle interactions, and help
solve the puzzle of why gravity, while perhaps most recognizable
to a lay observer, is the weakest of the fundamental forces.
The collider is a successor of
sorts to the Superconducting Supercollider, a high-energy
collider that was to have been built in Texas. The supercollider
was first proposed in 1983 and construction began in 1991, but
escalating cost estimates and other factors created controversy
and Congress cancelled the project in 1993, after about $2
billion had been spent.
UW scientists including Lubatti,
who initially worked on the Superconducting Supercollider, began
working on aspects of the Large Hadron Collider in the mid
1990s. The collider, which is to begin test operations in late
May or early June, will send hydrogen protons racing at nearly
the speed of light in opposite directions through parallel
underground cylinders that form a large circle about 16.5 miles
in circumference straddling the Swiss-French border. The
cylinders intersect at various points, allowing proton
collisions that produce subatomic particles that can be observed
by one of the six detectors, each positioned at one of the
intersections.
The Atlas detector contains more
than 430 chambers filled with aluminum tubes that range in
length from about 5 feet to 10 feet, each resembling a
fluorescent light tube. From the early 2000s to 2007, some
30,000 of the tubes were made at the UW and fitted into 80
chambers that were then packed into cargo containers and shipped
to Geneva. It cost about $50,000 to ship each chamber, and all
arrived undamaged. Another 60,000 tubes made with UW methods and
specifications were packed into chambers at two other U.S.
sites.
Once in Geneva, the chambers were
mounted into 32 sections shaped like giant pie wedges, which fit
together into two rings at either end of the main detector. The
last segment of the world's largest general-purpose particle
detector was lowered into place on leap day this year.
The tubes, critical to the
detector's work, have a skin just 1/64th of an inch thick. Each
has a gold-plated tungsten wire just half the width of a human
hair strung tautly through the center that will detect what
happens when subatomic particles collide at nearly the speed of
light.
The manufacture required great
precision, in some cases with tolerances of less than
one-thousandth of an inch, a tall order for instrument makers
and machinists in the UW Physics Department. A major part of
their success was designing and making the equipment that could
replicate such precision. Threading the tiny wires was another
great challenge.
"Maintaining that kind of
precision can be very difficult when you're working on scales of
more than 9 feet, but we were able to do it," Daly said. "We
found that students with good eyes were able to thread the wires
very easily. If I tried to do it, I couldn't even see the wire."
The other institutions that
worked on the manufacture of tubes for Atlas using techniques
and specifications developed at the UW are the University of
Michigan; the University of California, Irvine; Brookhaven
National Laboratory; and the Boston Muon Consortium, which
includes Harvard University, the Massachusetts Institute of
Technology, and Tufts, Boston and Brandeis universities.
In addition to Lubatti, other UW
physics participants include professors Tianchi Zhou and Paul
Mockett, who retired in 2005, and staff members David Forbush,
Joshua Wang and Matt Twomey. Participants from mechanical
engineering are Daly and lab engineer William Kuykendall.
Source
http://www.azom.com/news.asp?newsID=12308
Large Hadron Collider being constructed at CERN
Written by
YASSMIN ATEFI
Today,
many high-energy physicists believe that they
are continuing the same scientific thoughts that
began over 2,000 years ago in ancient Greece. It
was decided then that everything in the world
must me made up of tiny indivisible things
called atoms.
Only 100 years ago was the existence of atoms
proven, but it wasn't until the 1930s when
scientists were able to put down the basic
equations of quantum mechanics, so that even the
simplest atom - the Hydrogen atom - could be
understood.
Now, physicists have not stopped looking deeper
into the atom as the Large Hadron Collider
(LHC), a particle accelerator, is being pieced
together at CERN in Geneva and is due to be
started up later this year.
"This is the greatest engineering feat of all
mankind. It took the combined resources of
nearly all of the countries in the world and
thousand and thousands of scientists," said John
Conway, UC Davis professor of physics and
collaborator on the project. "People started
designing this in the early 1990s and only now
is it reaching completion."
A particle accelerator is a device used to study
the nucleus, or center, of an atom. It is
designed to take two beams of protons,
accelerate them to extremely high energies, and
then smash them together. By smashing the
protons together, scientists hope to find
smaller particles that have never been seen
before.
These smaller particles are known as quarks, the
fundamental building blocks of hadrons. Hadrons
are subatomic particles such as protons and
neutrons. In other words, quarks are fundamental
building blocks of matter than makeup hadrons.
"When the electrons get shot inside the nucleus,
they bounce out at very high angles sometimes,
[showing that] there were smaller things still
inside neutrons and protons, which we call
quarks," Conway said.
At this point, there are six known types of
quarks: up, down, charm, strange, top and
bottom. Every proton, a type of hadron, for
example, has three quarks inside of it: two up
quarks and one down quark. Since an up quark has
a charge of 2/3 and a down quark has a -1/3
charge, a proton would then contain a 1 charge.
Currently, the largest particle accelerator is
the Tevatron at Fermilab, located near Chicago.
According to Robin Erbacher, a UC Davis
associate professor of physics and member of the
LHC team, the Tevatron collision energies are at
about two tera-electron-volts (TeV), which is a
trillion electron-volts. Comparatively, the LHC
will eventually reach a collision energy of 14
TeV - seven times the energy of the Tevatron.
This means that the possibilities for
discovering new particles and interactions are
huge. The more energy the protons have in the
accelerator, the more collision energy available
to make new, heavy particles that have never
been created on Earth before.
The LHC is 17 miles in circumference and about
100 meters underground, spanning the border
between Switzerland and France. By comparison,
the Tevatron is four miles around. Two beams of
protons will travel in opposite directions
inside the circular accelerator, gaining energy
every time they go around.
According to the European Organization for
Nuclear Research, physicists will use the LHC to
recreate the conditions just after the Big Bang
by colliding the two beams head-on at very high
energy. Teams of physicists from around the
world will analyze the particles created in the
collisions using special detectors in a number
of experiments dedicated to the LHC.
As the two protons go around the accelerator,
they hit each other in the middle of the
detector where a mini explosion occurs and
hundreds of particles come out. The particles
are surrounded with layers of different
detectors, which can capture the particles that
come out, and measure their energies and
directions in the hope of reconstructing what
happened when the two protons collided with each
other.
One such detector is the Compact Muon Solenoid.
"The detector we built gives you the first
information on the particles coming out of the
collision, called a pixel detector," said
Conway.
Conway relates the pixel detector to the pixels
in a digital camera. The difference is that as
detectors tell what particles are passing
through, the pixels are larger than the ones in
a camera, and the detector can take 40 million
pictures per second.
A particle that the scientists at CERN hope to
discover is the Higgs Boson. It is proposed that
the Higgs Boson is how particles acquire
different masses.
"If this theory is right with the new experiment
at the LHC, we hope to answer the question: is
there a Higgs Boson, and if so, what does it
look like, is it one particle, or many?" Conway
said.
The accelerator was built for discovery, and
while theories of what will be discovered do
exist, what will emerge from the device is not
entirely known.
"In general, I would say it is too soon to tell
how the LHC will benefit humankind," Erbacher
said in e-mail interview. "We often don't know
how our discoveries will help us in the future
until we've made them. This is indeed a pure
research science."
YASSMIN
ATEFI can be reached at
science@californiaaggie.com.XXX
Source
http://www.californiaaggie.com/article/748
1 2 3 4 5 6 7 8 9 10 Newest
articles
|